In the three-and-a-half years since Sandvik acquired Artisan Vehicle Systems, the mining industry’s appetite and demand for battery-electric vehicle (BEV) solutions has grown exponentially.
From conversations that were concentrated to mainly North America and involved the trial of BEVs, the sector has moved on to discuss commercial, fleet-sized applications to be deployed across the globe.
Sandvik, itself, highlighted this in July with the award of its biggest BEV contract to date – a 20-strong equipment order from Foran Mining in Canada.
With this increased industry focus on underground electrification with the help of battery-electric equipment, the discussions around battery system safety have also stepped up in terms of both the number and complexity of conversations.
These are conversations Sandvik Mining and Rock Solutions’ Battery and Hybrid Electric Vehicles (BHEV) Business Unit is more than prepared to have, according to the unit’s VP Strategy and Commercial, Jakob Rutqvist.
“It’s on us as an early adopter to spread the learnings and help educate the industry in terms of battery safety and engaging with the stakeholders involved in the supply chain,” he told IM.
Sandvik, with the help of Artisan, has clocked up hundreds of thousands of operating hours in terms of BEVs in mining over the last decade or so.
This has been underwritten by the Artisan battery system architecture, which has been designed to move with both mining market demands and battery technology.
Brian Huff, Vice President of Technology for the BHEV business unit, expands on this: “We produce in-house battery systems, which gives us a lot of capability in terms of controlling the design. We can be much more reactive to the field in terms of making changes to the design, versus an OEM that is using batteries designed for automotive or industrial applications where those higher volumes tend to drive design decisions.
“We don’t have that diversion of focus; we do what is right for mining.”
Doing what is ‘right for mining’ has led to Sandvik investing in a state-of-the-art battery system facility in Camarillo, California, boasting 100 MWh of annual battery production capacity. This same facility acts as a training and customer visitor centre with a test ramp that has a 20% grade and a whole area for mucking on the property.
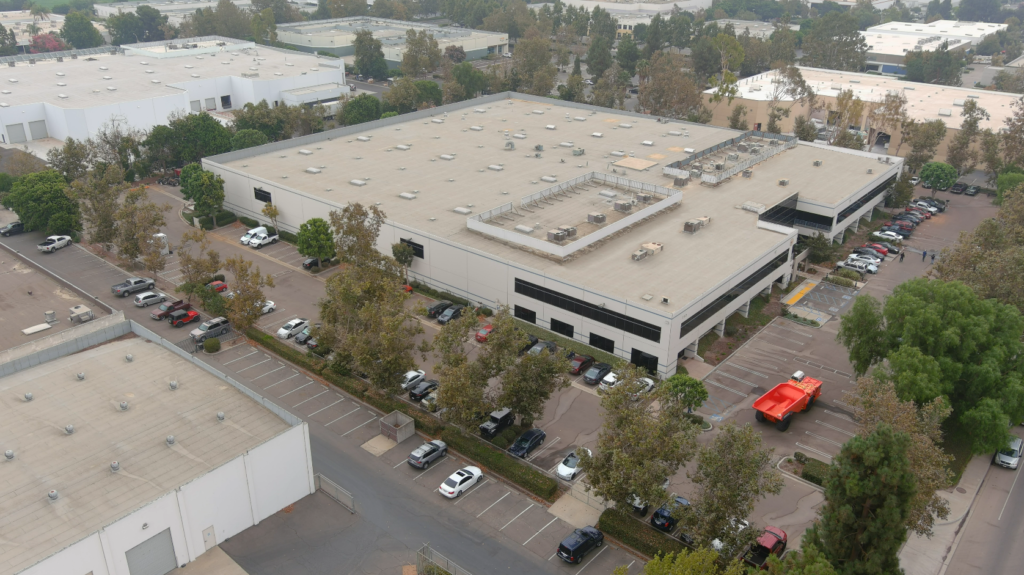
Huff said on the latter: “We will test every product that leaves the shop and do a lot of development work – our development cycle has, as a result, sped up.”
Beyond this, Sandvik’s mining sector commitment has seen the company invest in a battery system platform that – when it comes to safety – recognises the realities of operating machinery at underground mines.
Quality cells with the right chemistry
The inherent safety of Sandvik’s battery system starts at the cell level.
Artisan partnered with China-based CALB all the way back in 2015 as it looked to shore up a reliable and high-quality battery cell supply that could seamlessly fit into its battery system architecture.
Seven years on – half of that under the guise of Sandvik – Huff sees no reason to change.
“What matters from a battery system safety standpoint is consistency and high-quality cells,” he said. “That is achieved through high-volume manufacturing tied with automation and production controls that ensure the quality of production.
“CALB, which makes a lot of batteries for stationary and bus applications in China and globally, has all the compliance and testing completed on their cells and meet all the requirements from a safety standpoint.”
The battery cell manufacturer was also one of the early movers in the lithium iron phosphate (LFP)-based battery space, and Huff is keen to point out the safety benefits that come with using such battery chemistry.
“Our approach to battery safety, which is part of the standardised Sandvik approach for safety with ISO and other standardisation bodies, is to look, first, at reducing the severity of a potential incident or eliminating the hazard,” Huff said. “For us that means reducing the severity of a thermal runaway, which is primarily a chemistry choice.”
Thermal runaway is categorised as a chain reaction within a battery cell that occurs when the temperature inside a battery reaches the point that causes a chemical reaction to take place inside the battery. This chemical reaction produces heat, which drives the temperature higher, causing further chemical reactions to take place and further heat generation. Excessive heat generation at an accelerated rate can cause batteries to melt or be damaged beyond repair, or, in extreme circumstances, ignite and start fires.
With the potential to cause such an incident, thermal runaway preventions are often the first thing battery companies mention in safety briefings.
When plotting the main commercial battery chemistries against the heat-release-rate (HRR) on a graph, it is easy to see Huff’s point (see graph below). The rate of temperature rise (left) indicates the severity of a thermal event, with the higher the HRR, the harder it is to contain an incident, Sandvik says.
Taking all this into account, the LFP rate of temperature rise is over 100 times lower than other batteries with chemistries such as nickel-manganese-cobalt (NMC), lithium-cobalt oxide (LCO) and lithium-nickel-cobalt-aluminium (NCA), making containment more achievable, according to the company.
In thermal runaway tests, LFP-based cells have emitted a flammable gaseous electrolyte but do not self-ignite during standardised safety tests, Sandvik clarified.
Tests on batteries looking at the release of harmful emissions have also highlighted the safety benefits of using LFP-based batteries. A US CDC nail penetration test showed minimal emissions of the likes of carbon monoxide, nitrous oxides and hydrogen fluoride, compared with much higher levels emitted from NMC- and lithium-ion-manganese-oxide (LMO)-based batteries.
Passive and intrinsic safety
The battery chemistry choice fits into the ‘severity reduction’ basket in terms of safety controls, but it is not where Sandvik’s battery system safety approach ends.
“We then move to reducing the likelihood of the hazard occurring through design controls,” Huff said. “This is where the engineering comes in, with the best design controls often deemed to be passive and intrinsic – where you don’t have to do anything in the case an event arises.”
Sandvik has more than a handful of such controls in its locker, starting at the cell.
The cells in Sandvik’s battery systems are equipped with high-reliability vents that prevent pressure build-up in case of thermal runaway. This eliminates the risk of a case rupture/burst, according to the company. They also come equipped with a laser-welded aluminium housing that offers thermal conduction and mechanical safety with mylar and polycarbonate insulation.
A shutdown separator coating, meanwhile, is designed to melt if the temperature inside the cell reaches 110-130°C, blocking the ion flow, interrupting the current and preventing further temperature increases. An additional porous ceramic separator coating melts at 160-175°C, bonding with a polypropylene electrode separator to help block dendrites and provide structural support to prevent shrinkage and maintain the separation of electrodes.
Huff expands on this: “Dendrite growth is an effect of overcurrent, overcharging, charging at cold temperatures and a side effect of ageing. Excessive growth can result in an electrical short as well, which can then lead to thermal runaway.”
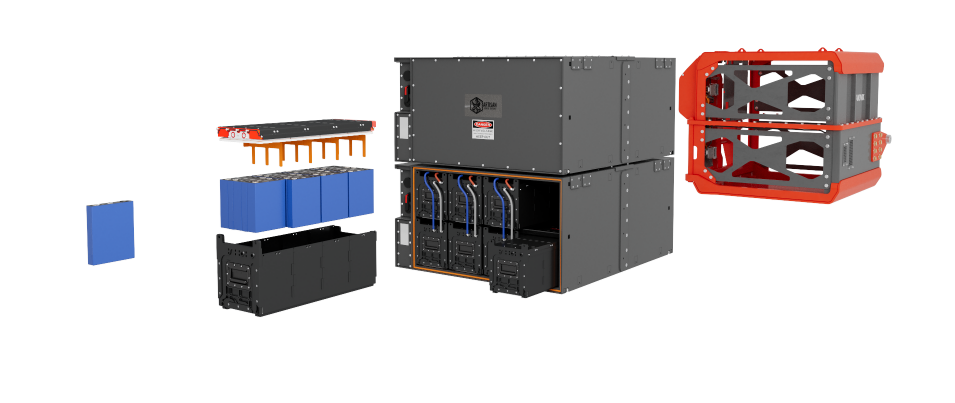
These cells are placed into a battery module, which operates at low voltage for safe servicing, comes with isolating foam potting to block moisture and prevent isolation faults, offers thermal conduction to draw heat away from a hot cell and distribute it across the entire module to moderate temperature rises, and offers environmental and mechanical damage protection, according to Sandvik.
These modules are then incorporated into a battery pack, which comes with mechanical protections such as a 6-mm plate steel enclosure; a non-conductive coolant to manage temperature during charging; and gore vents and drains to prevent pressure build-up, allowing vented gases to expel air in the enclosure and fluids to drain rather than collect, all while limiting ingress of dust and contaminants.
The redundant configuration of the battery pack contactors allows the circuit to be broken in the case of over/under voltage, isolation fault, over temperature or overcurrent, the company says. This may also be triggered by a high-voltage interlock loop (HVIL) system. Lastly, the inclusion of 600 A fuses provides protection against overcurrent and damage from external shorts.
Finally, this battery pack is enclosed in a cage that offers, Sandvik says, robust mechanical protection, mobility and swap-ability, plus quick access to the pack modules without cage disassembly.
Beyond design controls
“We start with the assumption that, however good, design controls should never be believed to be 100% effective,” Huff said. “You cannot just approach the issue by never considering the likelihood of thermal runaway, for instance. It is unrealistic, especially in a mining environment where accidents happen, damage occurs and mistakes in servicing can arise.”
This leads the company on to the active controls it includes in its battery systems.
“All this starts with monitoring,” Huff said. “It could be looking at temperatures – the case temperature, terminal temperature, as well as the temperature of the conductors – and voltage.”
The Battery System Controller (BSC) is responsible for protecting the battery – calculating the limits and thresholds, monitoring the HVIL, isolation, temperature and currents and connecting the battery and communicating the status – but it is not the system that implements the controls and limitations.
“The battery system controller communicates what the limits are – only 400 A in discharge due to heat, for example – but the master controller unit (MCU) is the one responsible for accomplishing those limits,” Huff explained. “If the MCU fails to do that – drawing too much current, for instance – then the battery system controller opens its connectors and disconnects power as a last resort.”
The battery monitoring system (BMS) monitors the cell voltage and temperature (case temperature, terminal temperature and conductor temperature, for instance), manages the cell balancing and communicates data to the BSC.
This monitoring will be further enhanced with the incorporation of Akkurate (a battery analytics company acquired by Sandvik earlier in the year) and its remote battery diagnostic and prognostic platforms into the BHEV business unit, with Rutqvist saying that “embedded” monitoring software on the battery and “remote health monitoring” applications are the first development priorities, post-integration.
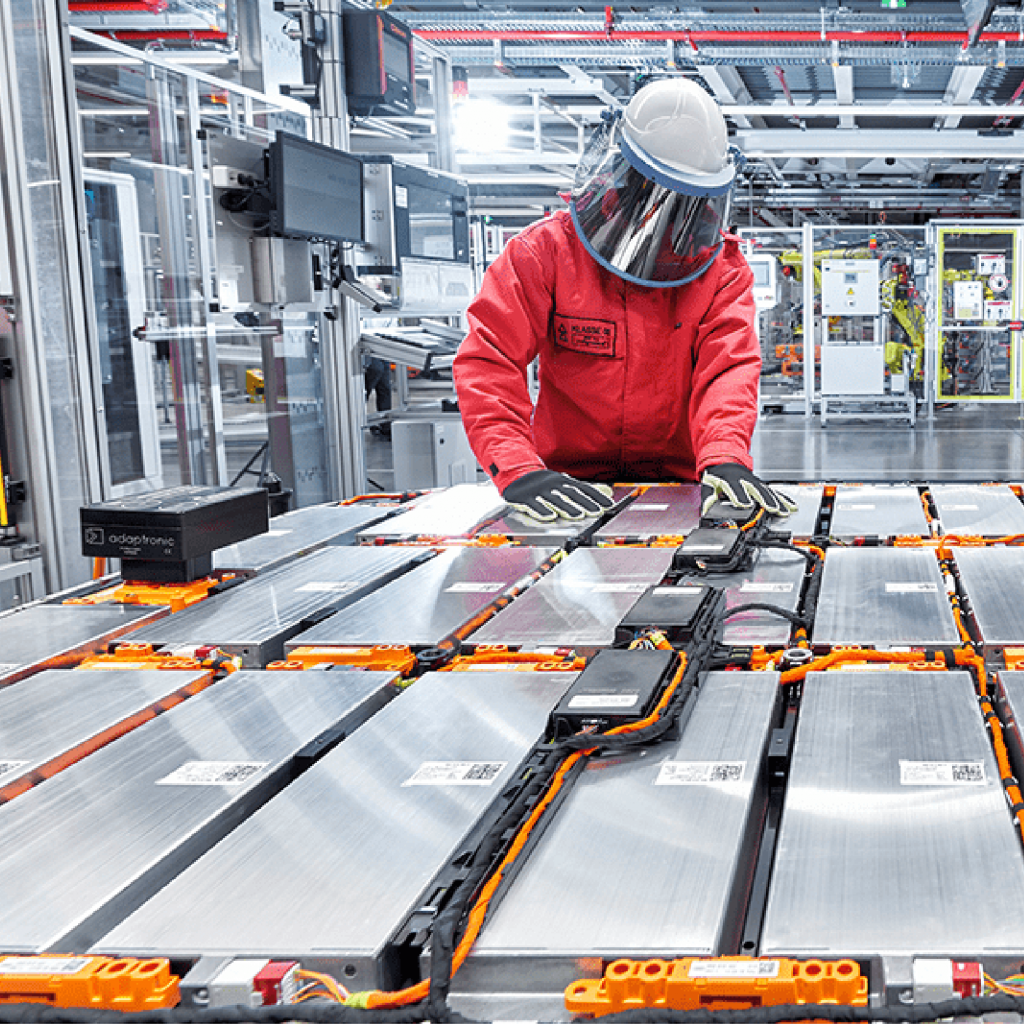
Such monitoring can go a long way in ensuring safety from within the system, but you cannot always limit external threats, Huff said.
“In terms of electrical failure modes, you can prevent overcharging and over-discharging through monitoring-based functions, but you can’t prevent deformation, mechanical damage or penetration from foreign objects,” he said.
This is where the ‘suppression’ element comes into play.
Sandvik uses a potassium-based, electrically non-conductive chemical emulsion for its fire suppression system. It acts by filling the battery pack interior with an aerosol agent that chemically interrupts combustion and stops a potential fire in its tracks.
“We’ve had a couple of incidents in the past, which were minor, and these suppression systems came in and did the job we prescribed for them,” Huff said. “They are not designed to put out a widespread battery fire, where, instead, the chemistry selection and the other passive controls we have designed in are the safety barriers.”
No expense spared
This three-step safety approach is indicative of the company’s focus on risk reduction for its customers, with Huff saying the company often looks beyond industry standards and regulations when designing features in.
“The volume and value proposition of our products change the battery system design requirements,” he said. “We’re not trying to save a dollar here by reducing the thickness of, say, the enclosure, or amending some design feature for cost effectiveness.
“Safety and reliability are way more important than the cost of manufacturing the system.”
There are plenty of examples of this safety-design-over-cost philosophy on board its battery systems.
For instance, the company has minimised the use of cables, designing as much as possible with busbars to avoid potential electrical shorting. All these busbars are powder coated and protected from an electrical perspective, meaning any “casual” contact from operators or service technicians will not result in the live conductors being engaged.
When it comes to isolation monitoring, the company has also designed in safety precautions.
“Isolation monitoring was originally mandated for battery systems to notify technicians about the potential for an electrical shock if there was an isolation fault,” Huff said.
“Beyond that, it can be used as an early warning for a higher current short circuit where an isolation fault occurs that is lower than a certain resistance.”
Huff and his team have carried out some research and chosen 5,000 ohms as the relevant threshold for this particular risk.
“If you have a 5,000-ohm isolation fault in a location and have a direct connection from another point to the chassis, you can create a short circuit with enough power to melt some of the protective materials in the battery system and potentially accelerate this to a much higher current short circuit,” he said.
This second isolation monitoring consideration requires a differentiated response.
“Shock hazards are a warning situation – you need to notify people there is a hazard and behave differently,” he said.
“You need to handle a short circuit risk – a low impedance isolation fault – differently. For us, it means shutting the machine down and isolating the fault through removing the modules or locating the fault and transferring the modules.”
With Artisan’s battery systems designed to be broken down into individual batteries and transported individually as needed, the company can do exactly this.
“There have been a few battery fires reported in mines over the last five or so years, involving different types of equipment,” Huff said. “The two I know about had nothing to do with a BMS response and everything to do with isolation faults. How you handle a battery with this issue is, therefore, a key consideration.”
Backward-compatible benefits
Huff, a co-founder of Artisan, can contextualise the mining proposition better than most considering his experience in both electrifying the automotive sector and heavy-duty commercial vehicles – two sectors Artisan served prior to shifting focus to underground mining.
“A key difference is the level of serviceability required,” he said. “Mining is a very different world; you are separated so much from a nice clean shop with all the facilities and space to do work. With cars, there is such easy infrastructure in place; mining is not like that.
“At mine sites, there is huge pressure to do the work in-situ with the tools you have in your backpack and, if you don’t have the specific tool, you are typically going to make the tool or do without it. You have to have a product designed for that environment, and that is exactly what we have done.”
Rutqvist said the standardisation and commodification of Sandvik’s battery systems comes at the cell level, which leaves the company open to adapt and customise according to mining industry demands.
“If you take the market at a battery system level, mining customers don’t count in thousands, they count in hundreds,” he said. “Our average customer is big, and they expect to be very close to us when it comes to the product and the product development; our largest customers are very big and expect to have a say in the development and the requirements on the battery system.
“We’re happy to be middlemen for the battery cell, but we don’t want to be middlemen for the battery system design.”
Over the past decade or so – and going forward – this has enabled the company to take advantage of battery technology developments as they happen.

Haley-Anna Blinn – currently a BEV Applications Specialist at the Sandvik BHEV business unit and previously an Electrical Engineer at the Macassa gold mine in Ontario, Canada, which has one of the biggest battery-electric fleets in the world – has been on the receiving end of this.
“So much is changing in the battery space all the time,” she said. “I have only been involved for five years, and I have seen a lot of change.
“We recognise that, so it is important we design our systems to accommodate future design changes or even battery chemistry improvements when it comes to energy density.
“With the older vintage of equipment, there was a change of cell supplier at one point that resulted in cells with a different form factor.
“While this changed the number of cells in a module based on their characteristics, it was a change that was carried out seamlessly when the cells were due to be refreshed. The module had a similar form factor, so was backward-compatible from a battery system design perspective.”
The new cells also ended up improving performance by about 20% or so, according to Blinn.
With the average battery cell life being 3-5 years, battery performance continuing to improve exponentially over a similar timeframe and the internal funding capabilities of the Sandvik Group, Sandvik BEV customers could be in line for similar step-change improvements in the future.
There are more subtle changes the company can make to its battery systems tailored to the operation at hand, too.
Leveraging sophisticated modelling software and a databank that goes back to the start of Artisan’s BEV journey, technicians can tweak the system at the factory to the conditions they will likely experience underground.
This goes beyond implementing a simple speed restriction to protect the battery system and the operators.
“Some mines might be more conducive to a slower charge than others based on the duty cycle, so we can configure these parameters at the factory,” Blinn explained. “Other mines may impose a temperature limit based on the operating conditions underground that goes beyond the standard limits we program. We can make those changes to ensure the system engages differently during operation.”
Having solved the obvious teething problems that came with introducing BEVs at underground mines over the last decade-or-so, Sandvik is moving into a consolidation phase where refinements to its system design will take place as opposed to major overhauls.
At a time when mining companies require improved performance and uptime from these machines to achieve their own electrification and productivity goals, the company’s mining-focused, safety-conscious battery system design philosophy continues to set it apart.